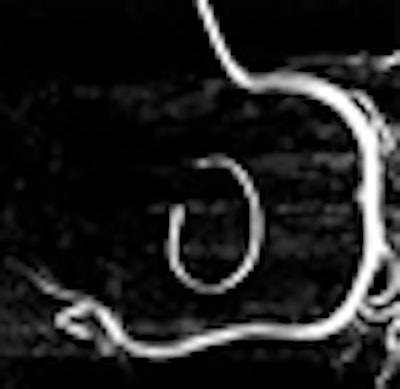
Researchers at the recent Computer Assisted Radiology and Surgery (CARS) meeting in Barcelona, Spain, presented new work that aims to simplify the difficult task of evaluating coronary artery images.
Researchers from Germany say their new automated phase-selection method yields sharper coronary artery reconstructions by mapping the centerline of each segment -- and rejecting phases whose centerlines stray too far from the mean.
To finish cleaning up the images, a motion-compensation technique then deforms the projections in each segment to line up the vessels in 3D. This step further minimizes blur, paving the way to better treatment planning and follow-up.
Three-dimensional reconstructions of the coronary arteries, based on electrocardiogram (ECG)-gated acquisition, offer great advantages over 2D mapping produced by gold-standard coronary angiography, said principal investigator Eberhard Hansis from Philips Healthcare research in Hamburg, and the University of Karlsruhe in Karlsruhe, Germany, in his presentation.
The quality of 3D reconstructions varies over the cardiac cycle. It is usually best near the end-systolic and end-diastolic rest phases, but the optimal phase cannot be predicted before the acquisition.
"Unfortunately, the optimal phase depends on heart rate; it also varies from patient to patient and varies [depending] on whether we are looking at the left or the right branch. So for each phase we need an automated method to detect the optimal recon phase," Hansis said.
Previous approaches to the phase-selection question include histogram-based automated techniques, and alternate methods based on choosing phases with the least motion in a single plane.
The proposed automated phase-selection process selects heart phases with minimum inconsistency of the motion state in selected projection data. A projection-based motion-compensation method then deforms all of the projections in order to overlay them in the 3D space. The combined techniques yielded consistently improved visualization of arteries compared to manually selected reconstruction phases.
While the researchers' advanced image-processing methods were applied to 3D rotational coronary angiography for purposes of the study, they could also be adapted to MDCT.
3D rotational acquisition
Coronary angiography images are acquired with 3D rotational angiography, which puts the patient's heart at the center of a conventional C-arm system, Hansis said. Once the arteries are opacified by contrast agent injection, images are acquired with the system rotating approximately 180° for seven seconds while acquiring 210 projections in a sequence spanning 5-10 heartbeats.
"From this rotational acquisition, 3D reconstructions of the coronary arteries can be produced for use in diagnosis, quantitative vessel assessment, or interventional planning, for example finding the optimal viewing angles for a lesion," Hansis explained. "To perform 3D reconstructions in one cardiac phase we select the projections belonging to that phase from the whole sequence by using ECG gating."
![]() |
ECG-gated rotational coronary angiography scans a patient's heart at the center of a conventional C-arm angiography system. The system rotates approximately 180° for seven seconds, acquiring approximately 210 projections over 5-10 heartbeats. All images courtesy of Eberhard Hansis. |
As in MDCT acquisition of coronary artery images, however, picking the wrong phase leads to blurred images and impaired diagnoses. The proposed method aims to optimize phase selection "by looking for the ones with the best consistency of the vessel centerline in 3D," Hansis said. "By this we mean that the angiograms should consistently show the coronary arteries in the same locations."
Automated phase selection
"Let's assume we have three projections belonging to one cardiac phase," Hansis proposed. "In each projection we calculate at each point the square distance to the closest centerline" of the artery segment. The resulting square-distance map is then back projected into a 3D reconstruction volume, he said.
Next, the contributions from each back projection are calculated to create a volume-distance map with a minimum value at the 3D position of the vessel centerline. In this manner, consistent projections yield low minimum values, while the measurements from inconsistent projections are higher, Hansis said. Thus, the centerline geometry of each vessel is used to detect phases with minimum inconsistency of the motion states to the corresponding angiograms.
"We repeat the whole process for all cardiac phases and then arrive at a curve [demonstrating] the inconsistency of the vessel centerlines over the cardiac cycle," he said. The minima of this curve represents the optimal reconstruction phase.
"We evaluated this method on 22 clinical cases," Hansis said. "For each case, two optimal phases, one in end-systole and one in late-diastole, were automatically projected and all of the cases were judged by three observers."
![]() |
Vessel centerlines are detected using a multiscale, curvature-based "vesselness" filter with subsequent directional maxima detection and hysteresis thresholding. Minima of inconsistency measurements are performed over all cardiac phases to select the optimum reconstruction phases. From each centerline map, a squared-distance transform is calculated by pixel-wise squaring a chamber-distance transform. The squared-distance transforms are then back projected onto a reconstruction volume to receive a volume-distance transform, in which points with the shortest mean-squared distance to the centerline in the angiograms have minimum value. The normalized minimum-value forward projections are then calculated and integrated over the vessel centerlines, yielding a number; the lowest numbers represent angiograms belonging to similar motion states. |
In all, 82% of the cases showed optimal image quality, 14% showed image quality slightly lower than optimum, and only 5% produced image quality considerably lower than optimum, Hansis said. In all 22 cases at least one of the two phases, either end-systole or late-diastole, showed optimal image quality, he said.
Motion compensation
Even if the optimal reconstruction phases are chosen, however, the heart's motion state isn't consistent in all angiograms due to factors such as residual heart motion, breathing, or imperfect gating. "These motion effects are, of course, stronger in nonoptimal reconstruction phases," Hansis added.
This is where the second method, 2D motion compensation, comes in. The method differs from previous motion-compensated reconstruction methods, which incorporate 3D motion information into the reconstruction itself. In the proposed method, reconstruction of motion-compensated angiograms can be performed with any reconstruction model; the authors chose a filtered back-projection technique for the study.
Like phase selection, the motion-compensation process begins with projections selected for the reconstruction of a single cardiac phase by ECG gating, Hansis said. A reference reconstruction is performed, and then a maximum-intensity forward projection is calculated onto each angiogram.
"Now we apply an elastic registration algorithm, which deforms the projection data such that the vessel centerlines match as well as possible," he said. "Here we use an iterative closest-point approach" using the vessel centerlines as marker points.
The transformation increases the consistency of each of the angiograms with respect to the coronary artery geometry, he said. The process is repeated for all projections. The resulting images reduce the influence of both noise and imperfect centerline filtering, yielding clearer reconstructions with less motion blur, Hansis said.
"We tried this method on a dynamic coronary heartbeat phantom that performs a beating motion," he said. The results showed improved accuracy in reconstructed vessel radii due to motion compensation.
![]() |
The projection motion-based compensation technique applies an elastic transformation algorithm to match forward-projected reconstructions of rotational coronary angiography images, reducing the effects of motion blur in reconstructions. |
According to these results, for a gating window of 40% the average error in reconstructed vessel radii dropped from 10.5% to 7.3% for the best phase and from 25.6% to 12% averaged over the entire cardiac cycle.
Robust
"This method proved to be robust and accurate in the evaluation of 22 clinical cases" as an optimal phase compensation method which looks for phases with the best centerline consistency, Hansis said.
![]() |
Reconstruction of a left coronary artery at four phase points in the cardiac cycle (top row, no motion correction [m.c.]), and reconstructions with motion compensation (bottom row). ES (end-systole) and LD (late-diastole) denote the rest phases of the cardiac cycle. The grayscale is identical for all images, which are maximum-intensity projections. Gating window: 40%, cosine-squared weighting. |
As for the second method, projection-based motion compensation, a key advantage is that it does not rely on a specific coronary artery model, so it can be easily integrated into existing reconstruction methods, he said. It is also fully automated and delivers improved image quality with less blur and far greater detail.
![]() |
Volume rendering at 68% RR created with automated phase selection and motion compensation. |
"The combination of these methods may lead to high-quality 3D coronary artery reconstruction," he said.
By Eric Barnes
AuntMinnie.com staff writer
July 14, 2008
Related Reading
64-slice MDCT valuable for assessing coronary artery disease, July 4, 2008
Global RV function on ECG-gated 64-slice MDCT compares well with MRI, July 4, 2008
CARS report: Fuzzy theory yields sharper CTA images, June 27, 2008
3D development outpaces facilities' readiness, June 23, 2008
Video game technology speeds beating-heart surgery, June 9, 2008
Copyright © 2008 AuntMinnie.com