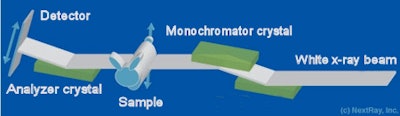
However, these tiny features can now be identified with a version of CT called diffraction-enhanced imaging, according to researchers at Brookhaven National Laboratory in Upton, NY, and the State University of New York (SUNY) in Stony Brook. What's more, this partnership says that its technique has the potential to deliver early diagnosis of Alzheimer's disease (NeuroImage, Vol. 46:4, pp. 908-914).
Alzheimer's disease, a disorder causing dementia in tens of millions of people worldwide, is caused by the buildup of dense areas of protein in the brain. These "plaques" contain a protein called amyloid beta and are just 5 to 200 µm in size.
Finding individual plaques
The U.S. team identified individual amyloid beta plaques in a mouse brain with the diffraction-enhanced imaging technique they first developed in 1995. These plaques have previously been observed with the same technique by Japanese researchers, who reported their results in 2006. However, on that occasion the brain tissue was dissected rather than being in a whole brain.
The diffraction-enhanced imaging tool used by the U.S. researchers employs x-rays generated from Brookhaven's National Synchrotron Light Source (NSLS). As this monochromatic beam passes through the sample, x-rays scatter and refract at different angles, depending on the tissue's characteristics. These differences are amplified with an analyzer crystal.
This crystal has a very narrow reflectivity profile and it produces a peak reflectance for x-rays unaffected by transmission through the sample. For deviations of just a few microradians, reflectivity drops to nearly zero. Thanks to the steep slope associated with this reflectivity profile, angular changes in the transmitted beam are converted into changes in intensity that are recorded on a detector array. By repeating this process over a wide variety of incident angles, it is possible to construct a 3D image.
![]() |
Image courtesy of medicalphysicsweb. |
Mapping changes in density
"With our technique, the 3D dataset represents a map of the changes in density," explains team member Dean Connor, a former researcher at Brookhaven who has recently moved to the University of North Carolina. "Anywhere there is an interface between two materials, there will be a dark or light spot in the 3D data," he explains.
The superior resolving power of the team's diffraction-enhanced imaging tool stems from a 1,000-fold increase in the intensity of the x-ray beam compared to that used for conventional tomography. "While diffraction-enhanced imaging does not have improved spatial resolution compared to normal x-ray imaging, it does generate significantly more contrast for soft-tissue features," says Connor. "This allows smaller soft-tissue features to be seen."
Using their imaging tool, Connor and his co-workers have identified amyloid beta plaques with a diameter of less than 30 µm. These had a difference in density from the surrounding brain tissue of just 2%.
The team compared these results with those it obtained by staining slices of brain tissue and identifying the plaques under a microscope. Good agreement was observed when comparing the size distribution of the plaques and their density.
Alternative imaging techniques
MRI can also image tissue, but it produces an inferior spatial resolution. "While MRI can allow for exquisite soft-tissue contrast, even high-field, high-resolution small-animal imaging systems have a resolution of 20 to 30 µm," explains Connor. In comparison, diffraction-enhanced imaging has a theoretical resolution of just 2 µm, although this would require a dose that exceeds the limit that can be given to patients.
However, a synchrotron source is a large and expensive facility not suitable for clinical use. Connor points out that if diffraction-enhanced imaging is to screen humans for Alzheimer's disease, then it will have to be implemented with a conventional x-ray tube. A spin-off company called NextRay is working toward this.
The Brookhaven-SUNY partnership also wants to improve the imaging system, so that it can reveal amyloid beta plaques through a mouse skull. In addition, the team wants to develop a new high-throughput, high-resolution imaging system that will be implemented at NSLS II, which is being built at Brookhaven and should offer a much brighter x-ray beam by 2015.
Benefits and obstacles
Alessandro Olivo, a researcher at University College London with expertise in CT, is very supportive of the work of Connor and his colleagues: "X-ray images have properties -- primarily of resolution -- which are not accessible by any other imaging technique."
However, he points out that there are many obstacles to overcome before x-rays can be used to screen humans for Alzheimer's disease.
This includes the development of a new generation of detectors that combine high-resolution with high-efficiency, to ultimately limit the dose given to the patient. "This resolution level also poses a problem in terms of data volumes and data analysis. This would have to be dealt with if a human brain is imagined, instead of that of a mouse."
By Richard Stevenson
Medicalphysicsweb contributing editor
August 6, 2009
Richard Stevenson is a science and technology journalist based in the U.K.
Related Reading
New x-ray technique images soft tissue, June 2, 2003
© IOP Publishing Limited. Republished with permission from medicalphysicsweb, a community Web site covering fundamental research and emerging technologies in medical imaging and radiation therapy.