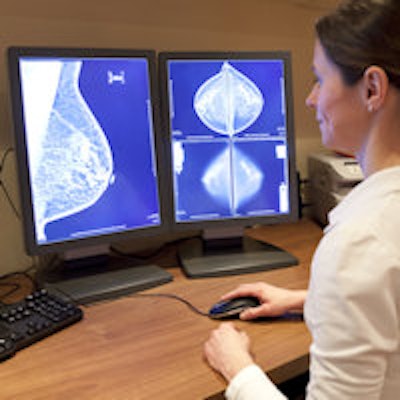
Although more than three-quarters of women strongly agree that getting regular mammography screening is important, just over half actually follow through, according to a study published in the September Journal of Women's Health. The study highlights a need for mammography awareness beyond just painting things pink.
The finding is discouraging in light of the fact that annual mammography screening has been shown to reduce breast cancer death by 19%, according to a team led by Dr. Susan Harvey, director of breast imaging at Johns Hopkins. Harvey's group conducted a survey that assessed women's knowledge of mammography's benefits and its coverage status under the Patient Protection and Affordable Care Act (ACA), as well as obstacles to it.
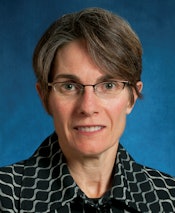
"Mortality from breast cancer has decreased significantly since 1990, probably related to improved mammography technology and detection, increased public awareness of the value of screening, and more effective treatments," the authors wrote. "Despite these improvements, breast cancer remains a serious health concern and is the second leading cause of cancer death among all women."
So why the disconnect? Key barriers include fear that a mammography screening exam will be expensive and won't be covered by insurance. This is unfortunate, since the ACA provides women with mammography screening at no cost -- a fact two-thirds of the women surveyed did not know, Harvey said.
"It didn't surprise us that cost is an important factor for women, or that most of the women who participated in our survey did not know that the ACA covers screening mammography," she told AuntMinnie.com. "Perhaps breast imaging centers could consider offering women information about ACA coverage along with information about the benefits of mammography."
Who knows what?
Under the auspices of the Society for Women's Health Research, Harvey's group administered the survey between September and October 2014 to 3,501 women. The women ranged in age from 18 to 93 years and spanned a variety of racial/ethnic groups (J Women's Health, September 2015, Vol. 24:9, pp. 777-783).
Seventy-eight percent of survey respondents agreed that mammography screening is important, but only 54% reported having an annual exam, the researchers found. Women with health insurance were two times more likely than those without to have annual mammography (57% versus 23%). In fact, 30% of uninsured women reported never having had a mammogram, compared with 10% of insured women.
Women older than 50 and those with a prior history of mammography demonstrated a slightly better knowledge of mammography than women in the 40 to 50 age group. Women younger than 50 and those without prior experience of mammography were less aware of ACA coverage, according to Harvey and colleagues.
"Younger women and those with no prior mammogram represent opportunities for educational outreach," they wrote.
As for motivating factors, 56% of the survey respondents said that a recommendation from their primary care provider and 53% said that a reminder or help scheduling a mammogram at their annual checkup were the greatest influences. Other motivating factors were personal medical history (40%), family history of breast cancer (38%), concern based on a self-exam (37%), and breast cancer awareness ads or information (33%).
The researchers also found some racial/ethnic differences in motivating factors; in particular, black and Hispanic women were almost twice as likely as white women to be motivated by breast cancer awareness information or family recommendation.
Reducing recalls
Almost half of the women who participated in the survey experienced being called back after an inconclusive mammogram; 89% of these recalls proved to be benign. Harvey's group found a marked emotional effect on women who were called back, especially those between the ages of 40 and 50: In this age group, 58% reported being scared and 57% reported being stressed by being recalled for more testing.
"The impact of fear and anxiety is no small matter for patients," the authors wrote. "It can affect family, friends, work, and other aspects of well-being, and could conceivably contribute to uncertainty about the accuracy and value of breast cancer screening."
Also, recalls are expensive, Harvey and colleagues noted. In 2010, the cost of callbacks in the U.S. was $1.6 billion. And the U.S. rate of recalls after inconclusive mammograms tends to be high -- more than double the rate in the U.K., for example.
"When we did our literature search, we were finding estimated U.S. recall rates as high as 15% -- and the guideline is 10% or less," Harvey told AuntMinnie.com. "Personally, I think 10% is too high. And as breast imagers we may think that it's 'just a recall,' but that's not how women feel. They're really frightened."
Johns Hopkins has reduced its own recall rate by gathering and evaluating recall data, re-examining callback cases, and determining the outcome of the recall. Finally, Johns Hopkins requires that two breast imagers must agree that a recall is necessary before it is made, according to Harvey.
"When we started this process, our group recall rate was around 11%," she said. "Now it's half that. Our goal is 5%, so we're getting close."
Beyond the pink
The study shows a continuing need to educate women about the cost of mammography and available insurance coverage, said co-author Christine Hughes of Hadley Hart Group in Chicago.
"Two-thirds of these women didn't know it wasn't going to cost them anything to get a screening mammogram [under the ACA]," she told AuntMinnie.com. "It's incumbent on hospitals to get this information out -- instead of just painting everything pink."